Abstract
Sawdust in Ethiopia, despite of its abundance, is not being properly utilized. Pre-treatment has been found to be crucial step before enzymatic hydrolysis of lignocellulosic resources to obtain sugars from such resources. Various pre-treatment methods have been developed to facilitate these bio-conversion processes. This research was aimed at investigating the effects of the combined pretreatments of steam and mild NaOH with white rot fungi (WRF) on sawdust samples from Eucalyptus globulus and Cupressus lusitanica were investigated. The amounts of losses of lignin, cellulose and hemicellulose contents of the pretreated sawdust samples were measured. Samples of the pretreated sawdust samples were then subjected to the enzymes from the hydrolytic wood rot fungi for hydrolysis into fermentable sugars. It was observed that lignin, cellulose and hemicellulose losses of the two sawdust types increased with the increasing incubation days but lignin and hemicellulose were preferentially degraded than cellulose. Sugar yield obtained from the sawdust pretreated in the combination of NaOH with WRF and steam with WRF was significantly higher compared to the yield obtained from the NaOH and steam alone pretreated sawdust samples. Pretreatment by the combination of NaOH with the wood rot fungi significantly modified the recalcitrance nature of the sawdust samples than when steam combined with the fungi. Similarly, with increasing enzymatic hydrolysis periods, sugar yields significantly increased. Higher sugar yields were obtained from sawdust samples of E. globulus than C. lusitanica. The ligninolytic and cellulolytic fungi tested resulted in a notable sugar yields indicating the possible application of the wood rot fungi in sugar production from lignocellulosic sawdust wastes which could be used for different industrial applications.
License
This is an open access article distributed under the Creative Commons Attribution License which permits unrestricted use, distribution, and reproduction in any medium, provided the original work is properly cited.
Article Type: Research Article
EUR J SUSTAIN DEV RES, Volume 4, Issue 1, 2020, Article No: em0110
https://doi.org/10.29333/ejosdr/6343
Publication date: 07 Dec 2019
Article Views: 1958
Article Downloads: 1220
Open Access HTML Content References How to cite this articleHTML Content
INTRODUCTION
Waste lignocelluloses, though largely underutilized, are the most abundant potential source for different industrial uses (Mishra et al., 2018). One of these is the requirement of fermentable sugars for large scale biofuel productions. Sawdust has been given attention as a feedstock for different industrial applications such as sugar productions over the past decade (Safarian & Unnthorsson, 2018; Trevorah & Othman, 2015). But the lignin-hemicellulose matrix surrounding the cellulose microfibrils prevents the microbial enzymes’ access to cellulose and inhibits its hydrolysis. This is usually solved by the removals or modifications of lignin and/or hemicelluloses (Amezcua-Allieri et al., 2017).
Alkali-based pretreatment involves the use of bases, such as mild NaOH, for the pretreatment of lignocellulosic feedstocks. It causes various structural alterations such as the depletion of lignin barrier and solvation of hemicelluloses (Ibrahim et al., 2011). NaOH pretreatment has also been observed to decrease cellulose crystallinity and the degree of polymerization (Rojo et al., 2013) thus increasing access for enzymes (Mirahmadi et al., 2010). On the other hand, steam has been implemented by using pressure to keep water in the liquid state at higher temperature to pretreat sawdust (Kamdem et al., 2015). It changes the biomass native structure by the removal of its hemicellulose content alongside transformations of the lignin structure, which make the cellulose more accessible for further enzymatic hydrolysis step (Alvira et al., 2010).
Combination of wood rot fungal pretreatment with other pretreatment methods has been reported to increase enzymatic hydrolysis yield (Alexandropoulou et al., 2017; Martín-Sampedro et al., 2017). A mild alkali pretreatment of corn stalks with I. lacteus for 15 days significantly facilitated lignin degradation by wood rot fungi (Yu et al., 2009). Fungal pretreatment of beech wood with P. chrysosporium and then steam explosion increased saccharification yield compared to a single pretreatment (Ma et al., 2010).
Ethiopia is categorized among countries with forest coverage of 10-30% (Reada & Beshah, 2018). Oromia regional state, for example, has high forest coverage of 3.1 million ha, which is 8.5% of its total land area (OFWE, 2014). Oromia forest and wildlife enterprise (OFWE) owned a total forest coverage of 1,752,488 ha, of which 74,215 ha, 1,209,955 ha and 468,318 ha are classified as plantations, natural forests and other lands, respectively. Oromia’s forest industry (OFI) has 31 sawmills located in different zones and it is the largest supplier of round wood and sawn timber in the country. While processing this huge potential very large amount of sawdust is being generated every year. Hence it is common to see a huge pile up by the vicinities of these sawmills (personal observations). Yet, the country is not utilizing this huge resource for better management and maximizes economic returns from forest industries.
Different authors reported different findings from Ethiopian fungi (Alemu, 2013; Belay et al., 2013; Bitew, 2010; Gebregiorgis, 2015; Hailu et al., 2012; Megersa et al., 2017c; Sahile et al., 2011; Teklebirhan & Bitew, 2015; Yenealem et al., 2013). But there is no effort has been done to produce sugars from lignocellulosic wastes through fungal enzymes except characterizing the enzymes from wood rot fungi (Megersa & Alemu, 2019; Megersa & Gure, 2018; Megersa et al., 2017a, 2017b, 2019). This research work was therefore conducted to evaluate the combined effect of NaOH and Steam pretreatments with wood rot fungal pretreatment on the hydrolysis of E. globulus and C. lusitanica sawdust samples.
MATERIALS AND METHODS
Fungal Cultures and Sawdust Sample Collections
Three WRF (Pholiota squarrosa 003-2G, Ganoderma aplanatum 006-2G and Polyporus giganteus 005-1G) were selected for the fungal pretreatment experiment based on their enzyme assays (Megersa et al., 2017a, 2017b). Three hydrolytic wood rot fungi (Phellinus tremulae 030-1D, Pholiota adipose 026-2D and Armilleria mellea 033-1G) were also selected for the hydrolysis experiment (Megersa & Gure, 2018).
Fresh sawdust samples of E. globulus and C. lusitanica were obtained from sawmills of Dagaga site, Oromia forest and wildlife enterprise (OFWE) in Ethiopia. These two tree species are the most widely utilized timber species in the country. The sawdust samples were dried, ground and passed through 5 mm pore size sieve.
Alkali Pretreatment
The sawdust samples were pretreated with alkali according to Mirahmadi et al. (2010). Six percent of NaOH (6%w/w) with a ratio of 1:10 w/v was used. The bottles were kept at room temperature for 2hrs by shaking at 150 rpm with the interval of 10 minutes. Following the pretreatment, samples were washed several times to neutral pH, filtered and solid mass collected for further use.
Steam Pretreatment
Sawdust (1:5 w/v in distilled water) was autoclaved for 2hrs (Shrestha, 2008). Following such pretreatment, mixture samples were washed several times to neutral pH, filtered and solid mass collected for further use.
Fungal Pretreatment
Both the alkali pretreated and steam pretreated sawdust samples were further pretreated by the WRF. Twenty grams of sawdust was placed in 100 ml Erlenmeyer flasks and conditioned with de-ionized water to moisture content of 75 %. The flasks were then autoclaved, cooled and inoculated with 10 ml homogenized liquid culture (Altaf et al., 2010) of each of the selected wood rot fungi on top of the substrate. The flasks were then incubated at 30 ºC in static conditions for 30, 45 and 60 days. Sawdust pretreated with either NaOH or steam was similarly incubated without fungal inoculation as control. After the predetermined incubation days, samples were agitated with distilled water (30 ml) at 180 rpm for an hour, and filtered under vacuum to remove the water-soluble components. The solid fractions were dried overnight in an oven at 65oC. Lignin, cellulose and hemicellulose contents of the two-way pretreated solid fractions were determined and the remaining samples were maintained for the enzymatic hydrolyses experiments.
Hydrolysis of the Pretreated Sawdust
Hydrolytic enzymes of the selected wood rot fungal isolates of Phellinus tremulae 030-1D, Pholiota adipose 026-2D and Armilleria mellea 033-1G were produced using the optimized medium of Hussain et al. (2012). The flasks were inoculated with 5 ml of each of the fungal inoculum and incubated at 30 oC for 12 days. After adjusting the pH to 5.0 with 50 ml of 0.05 M citrate buffer, the flasks were shaken for an hour on a rotary shaker at 150 rpm. The samples were then filtered through clean nylon cloth and the filtrates were centrifuged at 4000 rpm for 15 min. The supernatants were taken as crude enzyme extracts and stored at 4 °C until it was used for hydrolysis experiments.
Twenty grams of the two-way pretreated sawdust samples were added to each 100 ml flasks and autoclaved. After cooling, the flasks were loaded with crude enzyme extracts at 5% (v/w). A citrate buffer solution of 0.05 M was added to the flasks to achieve and maintain a pH of 5.0 (Trevorah & Othman, 2015). The flasks were sealed and held in at thermostat condition of 40 oC and manually shaked every 12 hrs. Aliquot samples were taken after 24, 48 and 72 hrs of incubation periods. The samples were then submerged in a water bath at 100 oC for 5 minutes, followed by an ice bath and centrifuged at 4000 rpm for 15 minutes. The supernatants were maintained for determining reducing sugar contents.
Composition Analysis
Klason lignin content of the two-step pretreated sawdust was determined according to Anonymous (Anonymous, 1997). Cellulose was determined according to Kurschner and Hoffer (1993) and hemicellulose was determined by weight differences. Total reducing sugar was determined by the 3,5-dinitrosalicyclic acid (DNS) method using glucose as the standard (Jadhav et al., 2013).
Statistical Analysis
The effects of combination of pretreatments on lignin, cellulose and hemicellulose reduction, the effects of fungal enzymes on the hydrolysis of the pretreated sawdust were evaluated using SPSS software for analysis of variance and significance tests at 95% confidence level. Normality and homogeneity of variances were checked before the actual analysis. Tukey simultaneous test was performed to assess statistical differences between treatments means of the triplicate measurements when statistically significant differences (p<0.05) were observed.
RESULTS AND DISCUSSION
Effects of Combined Pretreatments
The amount of lignin and structural polysaccharide losses of the E. globulus and C. lusitanica sawdust samples during NaOH-WRF and steam-WRF pretreatments were significantly increased with the increasing incubation days (p<0.05). We need to know the weight of the sawdust mass residue after pre-treatment of lignocelluloses since it is an indicator of the composition of the materials and the effect of the pretreatment method used. Figures 1 to 4 showed that the trend of mass loss when sawdust samples were subjected to steam and then to WRF and similarly to NaOH and then to WRF at various time intervals. Pretreatment experiments indicated that there was a decline in the sawdust mass recovered with increase in pre-treatment time and also an increased loss of lignin, cellulose and hemicellulose as a function of pre-treatment times. A higher pre-treatment time resulted in a higher removal of components. In both sawdust types, it was observed that pretreatment with NaOH followed by WRF showed higher lignin loss than when pretreated with steam and followed by WRF. It could be clearly seen that NaOH better facilitated the sawdust than steam for lignifying efficiency of the WRF.
NaOH-WRF pretreatment
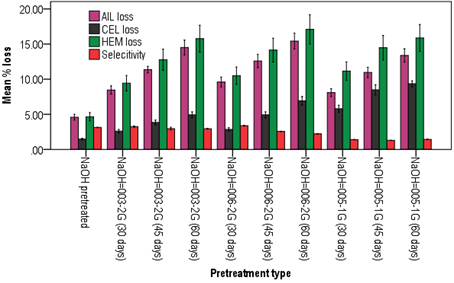
The highest lignin loss (15.43%) was observed when E. globulus sawdust pretreated with NaOH-G. aplanatum 006-2G for 60 days (Figure 1). The next highest lignin loss (14.51%) was obtained during pretreatment of the sawdust by NaOH-P. squarrosa 003-2G for 60 days. Unlike the endophytic fungi where degradation of lignin components within 28 days, the saprophytes take longer time in degradation of the lignin content on E. globulus (Martín-Sampedro et al., 2017). Relatively low lignin loss of 11.73% was seen during C. lusitanica pretreatment with NaOH-P. squarrosa 003-2G (Figure 2) which is lower than the loss of E. globulus. The low amount of lignin degradation by P. squarrosa and other WRF could be attributed to the high content of extractive substance contents in the cupressus and the eucalyptus species. Even though these two species of fungi degrade highly the lignin components, they also degrade the hemicellulose with the same percentage. Enzymes produced in the hydrolyzing of the hemicellulose eventually attack the cellulose to a lesser extent. The highest cellulose loss of 9.35% recorded when pretreated with NaOH-P. giganteus 005-1G for 60 days. On the other hand, the highest hemicelluloses loss of 17.10% was recorded when pretreated with NaOH- G. aplanatum 006-2G for 60 days. Martín-Sampedro et al. (2017) reported that lignin degrading WRF also associated to varying degree of sugar consumption. Thus low selectivity index for G. aplanatum fungi indicates for unsuitability of the fungi for the pretreatment of eucalyptus and cupressus species as it degrade the hemicellulose and eventually the cellulose components of the biomass.
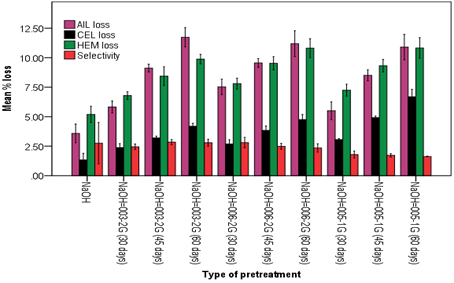
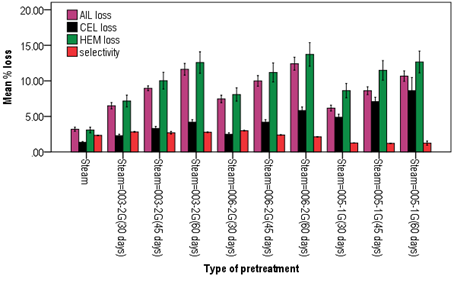
Steam-WRF pretreatment
In all pretreatments, the highest lignin, cellulose and hemicellulose losses were recorded during the 60 day of incubation period. Statistically insignificant lignin amount losses of 9.71%, 9.68% and 9.11% were recorded when E. globulus sawdust was pretreated with steam-P. squarrosa 003-2G, steam-G. aplanatum 006-2G and steam-P. giganteus 005-1G, respectively (Figure 3). During E. globulus sawdust pretreatment with steam- P. giganteus 005-1G, the highest cellulose amount of 8.64% was lost. On the other hand, the highest hemicellulose loss of 13.72% was observed during steam-G. aplanatum 006-2G pretreatment of the sawdust. In general, results showed that combined pretreatment of NaOH-WRF had better effect on E. globulus sawdust than steam-WRF resulting in better lignin losses.
In comparison with sawdust of E. globulus, lower lignin loss was observed in C. lusitanica. Lignin loss of 11.73% was seen during C. lusitanica pretreatment with NaOH-P. squarrosa 003-2G (Figure 4). The highest cellulose loss of 6.69% and hemicellulose loss of 10.83 % were recorded when pretreated with NaOH-P. giganteus 005-1G for 60 days.
Zhang et al. (2016) pretreated popular wood with the WRF T. versicolor C6915 for 8 weeks and then pretreated further with hydrothermal at 80, 100, 120, and 140 oC for 2 hrs which led to lignin degradation of 5.4%, 6.5%, 8.1%, and 8.8%, respectively. Our three WRF showed better ligninolytic efficiency over T. versicolor reported here displaying more lignin contents. It seems that pretreating with chemo-physical methods first and then with WRF like what has been done in this work than with WRF and followed by others. Alexandropoulou et al. (2017) conducted a combined pretreatment with white rot fungus Abortiporus biennis and NaOH and reported a significant effect on the lignocellulotic content of the biologically pretreated willow sawdust, exhibiting a considerable loss of lignin, cellulose, and hemicellulose which were of course similar to our findings. Combined pretreatments of both white rot fungi combined with NaOH and white rot fungi combined with steam, in this experiment also similarly resulted in a significant loss of lignin and polysaccharides compared the control results.
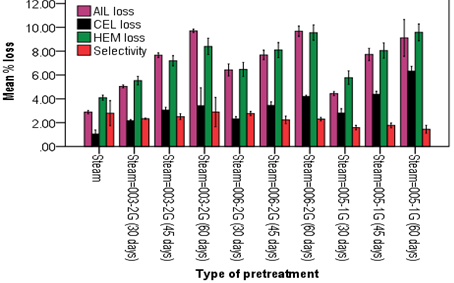
Enzymatic Hydrolysis of the Two-way Pretreated Sawdust Samples
Sugar yields in all the two-way pretreated cases were significantly higher than the sugar yields from the NaOH pretreated alone or steam pretreated alone sawdust samples. This indicates that the two-way pretreatments efficiently modified the cell wall structure of the sawdust samples giving better access for enzymatic hydrolysis. Results also indicated that the increasing enzymatic hydrolysis period resulted in a significantly higher sugar yield.
Enzymatic hydrolysis of NaOH-WRF pretreated of E. globulus sawdust: Three top, but with no significant differences (P≤0.005), sugar yields of 7.78 g/l, 7.54 g/l and 7.51 g/l were obtained from the NaOH-G. aplanatum 006-2G, NaOH-P. squarrosa 003-2G and NaOH-P. giganteus 005-1G pretreated E. globulus sawdust, respectively, all after 72 hrs of hydrolysis by enzyme extract from isolate 033-1G (Table 1). Hydrolytic enzyme from isolate P. adipose 026-2D yields higher sugar than the other two hydrolytic fungal isolates when NaOH combined with the three ligninolytic fungal isolates. Hydrolytic effect of enzyme from fungal isolate P. tremulae 030-1D also gave similar amount sugar yield. In all cases hydrolysis at 72 hrs incubation period was found effective.
Table 1. Sugar yields from the enzymatic hydrolysis of the NaOH-WRF pretreated E. globulus sawdust
Note: Means followed by the same letter are not significantly different at 5% level of significance. NaOH = NaOH pretreated sawdust; NaOH-003-2G = sawdust first pretreated with NaOH and then by P. squarrosa; NaOH-006-2G = sawdust first pretreated by NaOH and then by G. aplanatum; NaOH-005-1G = sawdust first pretreated by NaOH and then by P. giganteus; 030-1D, 026-2D and 033-1G are sources of hydrolytic enzymes from P. tremulae, P. adipose and A. mellea, respectively |
Enzymatic hydrolysis of NaOH-WRF C. lusitanica sawdust: With NaOH-WRF pretreated C. lusitanica sawdust, significantly different sugar yields were observed after enzymatic hydrolysis (Table 2). The highest sugar yield of 7.46 g/l was obtained from the NaOH-G. aplanatum 006-2G pretreated and then hydrolyzed with enzyme from isolate A. mellea 033-1G for 72 hrs. The remaining hydrolytic enzymes also gave slightly lower sugar yields but in considerable amount. The result also showed that sugar yields from the NaOH-WRF pretreated and enzymatically hydrolyzed sawdust of C. lusitanica was slightly lower than the sugar amount obtained from similarly pretreated and hydrolyzed sawdust of E. globulus.
Table 2. Sugar yields from the enzymatic hydrolysis of the NaOH-WRF pretreated C. lusitanica sawdust
Note: Means followed by the same letter are not significantly different at 5% level of significance. NaOH = NaOH pretreated sawdust; NaOH-003-2G = sawdust first pretreated with NaOH and then by P. squarrosa; NaOH-006-2G = sawdust first pretreated by NaOH and then by G. aplanatum; NaOH-005-1G = sawdust first pretreated by NaOH and then by P. giganteus; 030-1D, 026-2D and 033-1G are sources of hydrolytic enzymes from P. tremulae, P. adipose and A. mellea, respectively |
Enzymatic hydrolysis of Steam-WRF pretreated E. globulus sawdust: In general, Steam-WRF pretreated and enzymatically hydrolyzed sawdust of E. globulus sawdust gave lower than the sugar yield pretreated with NaOH-WRF (Table 3). Of Among all pretreatments of Steam-G. aplanatum 006-2G and Steam-P. squarrosa 003-2G of E. globulus sawdust, the two highest sugar yields of 6.83 g/l and 6.65 g/l were generated when hydrolyzed with enzyme from A. mellea 033-1G.
Table 3. Sugar yields from the enzymatic hydrolysis of the Steam-WRF pretreated E. globulus sawdust
Note: Means followed by the same letter are not significantly different at 5% level of significance. Steam = steam pretreated sawdust; Steam-003-2G = sawdust first pretreated with steam and then by P. squarrosa; Steam-006-2G = sawdust first pretreated by steam and then by G. aplanatum; Steam-005-1G = sawdust first pretreated by steam and then by P. giganteus; 030-1D, 026-2D and 033-1G are sources of hydrolytic enzymes from P. tremulae, P. adipose and A. mellea, respectively |
Enzymatic hydrolysis of Steam-WRF pretreated C. lusitanica sawdust: Similarly, the highest sugar yield of 6.62 g/l was obtained when sawdust of C. lusitanica pretreated by steam-G. aplanatum 006-2G and hydrolyzed with enzyme of isolate A. mellea 033-1G for 72 hrs (Table 4). Of the three pretreatment techniques employed on the two sawdust types, Steam-WRF pretreated C. lusitanica sugar yield were found to be the least.
Increased enzymatic digestibility from combined treatments can be attributed to a combined effect of the treatments. Similar reports were made by different scholars. Combined white rot fungus and alkali pretreatment at near room temperature cut down the time needed more significantly leading to higher efficiency of saccharification (Zhong et al., 2011). With a combination of hydrothermal and WRF Trametes versicolor C6915 treatment of popular, the sugar yields were higher (25.5%-51.1%) compared to white-rot only treatment (11.5%-28.6%) (Zhang et al., 2016). Qiang et al. (2010) developed a two-step liquid hot water pretreatment with the objective of achieving complete hydrolysis of Eucalyptus grandis and reported the maximum sugar yield during the 72 hrs hydrolysis periods.
Table 4. Sugar yields from the enzymatic hydrolysis of the steam-WRF pretreated C. lusitanica sawdust
Note: Means followed by the same letter are not significantly different at 5% level of significance. Steam = steam pretreated sawdust; Steam-003-2G = sawdust first pretreated with steam and then by P. squarrosa; Steam-006-2G = sawdust first pretreated by steam and then by G. aplanatum; Steam-005-1G = sawdust first pretreated by steam and then by P. giganteus; 030-1D, 026-2D and 033-1G are sources of hydrolytic enzymes from P. tremulae, P. adipose and A. mellea, respectively |
CONCLUSION
The purpose of this study was to determine the sugar yields of sawdust of E. globulus and C. lusitanica by integrating different pretreatment strategies. Combination of NaOH and steam with WRF improved the hydrolysis yields of the sawdust samples. The results showed that significantly higher sugar yields (p<0.05) were obtained from the combined treatments when compared to NaOH or steam alone pretreatments. With combination of mild NaOH and steam with WRF, lignocellulosic wastes could be efficiently converted to usable forms such as reducing sugars that could be used for different industrial applications. Hence the conversion of sawdust into sugar monomers could contribute toward the energy generation and serve as a sustainable solid waste management strategy. We recommend similar research works to be carried out to bring more efficient ligninolytic and hydrolytic wood rot fungi for such applications.
ACKNOWLEDGEMENT
The authors would like to thank the Ethiopian environment and forest research institute (EEFRI) and the Wondo Genet College of forestry and natural resources (WGCF-NR) for their financial support and laboratory facilities.
References
- Alemu, F. (2013). Assessment of wild mushrooms and wood decaying fungi in Dilla University, main campus, Ethiopia. International Journal of Advanced Research, 1(8), 458-467.
- Alexandropoulou, M., Antonopoulou, G., Ntaikou, I., & Lyberatos, G. (2017). Fungal pretreatment of willow sawdust with Abortiporus biennis for anaerobic digestion: Impact of an external nitrogen source. Sustainability, 9, 130. https://doi.org/10.3390/su9010130
- Altaf, S. A., Umar, D. M., & Muhammad, M. S. (2010). Production of xylanase enzyme by Pleurotus eryngii and Flamulina velutipes grown on different carbon sources under submerged fermentation. W. Appl. Sci. J., 8, 47-49.
- Alvira, P., Tomás-Pejó, E., Ballesteros, M., & Negro, M.J. (2010). Pretreatments technologies for an efficient bioethanol production process based on enzymatic hydrolysis: A review. Bioresour. Technol., 101, 4851-4861. https://doi.org/10.1016/j.biortech.2009.11.093
- Amezcua-Allieri, M. A., Durán, T. S., & Aburto, J. (2017). Study of chemical and enzymatic hydrolysis of cellulosic material to obtain fermentable sugars. Journal of Chemistry, Article ID 5680105. https://doi.org/10.1155/2017/5680105
- Anonymous. (1997). Standard test method for acid insoluble Lignin (Klason lignin) in wood. USA, ASTM D 1106-56.
- Belay, Z., Vestberg, M., & Assefa, F. (2013). Diversity and abundance of arbuscular mycorrhizal fungi associated with acacia trees from different land use systems in Ethiopia. Afr. J. Microbiol. Res., 7(48), 5503-5515. https://doi.org/10.5897/AJMR2013.6115
- Bitew, A. (2010). Antibactrial and antifungal activities of culture filtrate extracts of Pyrofomes demidoffii (Basidiomycete). World Applied Sciences Journal, 10(8), 861-866.
- Gebregiorgis, T. (2015). Antioxidant and antiinflammatory properties of cultivated mushrooms grown in Mekelle city, Tigray, Ethiopia. International Journal of Nutrition and Food Sciences, 4(5), 578-583. https://doi.org/10.11648/j.ijnfs.20150405.20
- Hailu, T., Selvaraj, T., Mengistu, L., & Negery, M. (2012). Evaluation of some native entomopathogenic fungi against pink stem borer (Sesamia calamistis Hampson) (Lepidoptera: Noctuidae) in sugarcane. Journal of Agricultural Technology, 8(2), 515-536.
- Hussain, A., Shrivastav, A., Jain, S. K., Baghel, R. K., Rani, S., & Agrawale, M. K. (2012). Cellulolytic enzymatic activity of soft rot filamentous fungi Paecilomyces variotii. Advances in Bioresearch, 3(3), 10-17.
- Ibrahim, M. M., El-Zawawy, W. K., Abdel-Fattah, Y. R., Soliman, N. A., & Agblevor, F. A. (2011). Comparison of alkaline pulping with steam explosion for glucose production from rice straw. Carbohydrate Polymers, 83, 720-726. https://doi.org/10.1016/j.carbpol.2010.08.046
- Jadhav, A. R., Girde, A. V., More, S. M., More, S. B., & Khan, S. (2013). Cellulase production by utilizing agricultural wastes. Res. J Agri Fores Sci, 1(7), 6-9.
- Kamdem, I., Jacquet, N., Tiappi, F. M., Hiligsmann, S., Vanderghem, C., Richel, A., Jacques, P., & Thonart, P. (2015). Comparative biochemical analysis after steam pretreatment of lignocellulosic agricultural waste biomass from Williams Cavendish banana plant (Triploid Musa AAA group). Waste Management and Research, 33(11), 1022-1032. https://doi.org/10.1177/0734242X15597998
- Kurschner, K., & Hoffer, A. (1993). Cellulose and cellulose derivative. Fresenius J Anal Chem, 92(3), 145-154.
- Ma, F., Yang, N., Xu, C., Yu, H., Wu, J., & Zhang, X. (2010). Combination of biological pretreatment with mild acid pretreatment for enzymatic hydrolysis and ethanol production from water hyacinth. Bioresour Technol., 101, 9600-9604. https://doi.org/10.1016/j.biortech.2010.07.084
- Martín-Sampedro, R., López-Linares, J. C., Fillat, U., Gea-Izquierdo, G., Ibarra, D., Castro, E., & Eugenio, M. E. (2017). Endophytic fungi as pretreatment to enhance enzymatic hydrolysis of Olive tree pruning. BioMed Research International, Article ID 9727581. https://doi.org/10.1155/2017/9727581
- Megersa, S., & Alemu, M. (2019). Qualitative assays and quantitative determinations of xylanolytic enzymes of wood rot fungi from Dagaga and Gambo forests, Ethiopia. Journal of Bioscience and Biotechnology Discovery, 4(4), 69-78. https://doi.org/10.31248/JBBD2019.101
- Megersa, S., & Gure, A. (2018). Qualitative assays and quantitative determinations of cellulolytic enzymes of wood rot fungi. American-Eurasian J. Agric. and Environ. Sci., 18(6), 347-357. https://doi.org/10.5829/idosi.aejaes.2018.347.357
- Megersa, S., Feleke, S., Alemu, M., & Gure, A. (2019). Fungal pretreatment and hydrolysis of sawdust wastes from Ethiopian sawmills for sugar production. World Applied Sciences Journal, 37(5), 438-445. https://doi.org/10.5829/idosi.wasj.2019.438.445
- Megersa, S., Gure, A., Alemu, M., & Feleke, S. (2017a). Qualitative assays and quantitative determinations of laccases of white rot fungi from plantation and natural forests of Arsi forest enterprise, Ethiopia. WSN, 67(2), 303-323.
- Megersa, S., Gure, A., Feleke, S., & Alemu, M. (2017b). Characterization of MnP and LiP from white rot fungi of plantation and natural forests of Arsi forest enterprise. IJPSS, 7(5), 6-25.
- Megersa, S., Gure, A., Feleke, S., & Alemu, M. (2017c). Macrofungi Species richness and diversity in Dagaga and Gambo plantation and natural forests of Arsi forest enterprise, Oromia, Ethiopia. Imperial Journal of Interdisciplinary Research, 3(1), 1681-1686.
- Mirahmadi, M., Kabir, M. M., Jeihanipour, A., Karimi, K., & Taherzadeh, M. J. (2010). Alkaline pretreatment of spruce and birch to improve bioethanol and biogas production. BioResources, 5(2), 928-938.
- Mishra, S., Singh, P. K., Dash, S., & Pattnaik, R. (2018). Microbial pretreatment of lignocellulosic biomass for enhanced biomethanation and waste management. 3 Biotech., 8, 458. https://doi.org/10.1007/s13205-018-1480-z
- OFWE (Oromia Forest and Wildlife Enterprise). (2014). Oromia Forest Industry (Brochure), Addis Ababa, Ethiopia.
- Qiang, T., Yu, Q., Zhuang, X., Yuan, Z., Wang, Q., Qi, W., … Xu, H. (2010). Two-step liquid hot water pretreatment of Eucalyptus grandis to enhance sugar recovery and enzymatic digestibility of cellulose. Bioresour Technol., 101, 4895-4899. https://doi.org/10.1016/j.biortech.2009.11.051
- Reda, H., & Beshah, B. (2018). Oromia forest industry’s supply chain network performance analysis. International Journal of Mechanical Engineering and Applications, 6(6), 142-149. https://doi.org/10.11648/j.ijmea.20180606.11
- Rojo, E., Alonso, M. V., Dominguez, J. C., Saz-Orozco, B. D., Oliet, M., & Rodriguez, F. (2013). Alkali treatment of viscose cellulosic fibers from eucalyptus wood: structural, morphological, and thermal analysis. J. Appl. Polymer Sci., 130(3), 2198-2204. https://doi.org/10.1155/2015/284250
- Safarian, S., & Unnthorsson, R. (2018). An Assessment of the Sustainability of lignocellulosic bioethanol production from wastes in Iceland. Energies, 11, 1493. https://doi.org/10.3390/en11061493
- Sahile, S., Sakhuja, P. K., Fininsa, C., & Ahmed, S. (2011). Potential antagonistic fungal species from Ethiopia for biological control of chocolate spot disease of faba bean. African Crop Science Journal, 19(3), 197-209.
- Shrestha, P. (2008). Enhanced bioprocessing of lignocellulose: Wood-rot fungal saccharification and fermentation of corn fiber to ethanol (Graduate Thesis and Dissertation). Paper 11492, 168pp.
- Teklebirhan, G., & Bitew, A. (2015). Profile of dermatophyte and non dermatophyte fungi in patients suspected of dermatophytosis. American Journal of Life Sciences, 3(5), 352-357. https://doi.org/10.11648/j.ajls.20150305.13
- Trevorah, R. M., & Othman, M. Z. (2015). Alkali pretreatment and enzymatic hydrolysis of Australian timber mill sawdust for biofuel production. J. Renewable Energy, Article ID 284250. https://doi.org/10.1155/2015/284250
- Yenealem, T., Muleta, D., & Woyessa, D. (2013). Mushroom consumption habits of Wacha Kebele residents, southwestern Ethiopia. Global Research Journal of Agricultural and Biological Sciences, 4(1), 6-16.
- Yu, J., Zhang, J., He, J., Liu, Z., & Yu, Z. (2009). Combinations of mild physical or chemical pretreatment with biological pretreatment for enzymatic hydrolysis of rice hull. Bioresour Technol., 100(2), 903-908. https://doi.org/10.1016/j.biortech.2008.07.025
- Zhang, L., You, T., Zhou, T., Zhang, L., & Xu, F. (2016). Synergistic effect of white-rot fungi and alkaline pretreatments for improving enzymatic hydrolysis of poplar wood. Indus. Crops and Products, 86, 155-162. https://doi.org/10.1016/j.indcrop.2016.03.041
- Zhong, W., Yu, H., Song, L., & Zhang, X. (2011). Combined pretreatment with white rot fungus and alkali near room-temperature for improving saccharification of corn stalks. BioResources, 6(3), 3440-3451.
How to cite this article
APA
Megersa, S., & Feleke, S. (2020). Enzymatic Hydrolysis of Two-way Pretreated Sawdust of Eucalyptus globulus and Cupressus lusitanica. European Journal of Sustainable Development Research, 4(1), em0110. https://doi.org/10.29333/ejosdr/6343
Vancouver
Megersa S, Feleke S. Enzymatic Hydrolysis of Two-way Pretreated Sawdust of Eucalyptus globulus and Cupressus lusitanica. EUR J SUSTAIN DEV RES. 2020;4(1):em0110. https://doi.org/10.29333/ejosdr/6343
AMA
Megersa S, Feleke S. Enzymatic Hydrolysis of Two-way Pretreated Sawdust of Eucalyptus globulus and Cupressus lusitanica. EUR J SUSTAIN DEV RES. 2020;4(1), em0110. https://doi.org/10.29333/ejosdr/6343
Chicago
Megersa, Shasho, and Sisay Feleke. "Enzymatic Hydrolysis of Two-way Pretreated Sawdust of Eucalyptus globulus and Cupressus lusitanica". European Journal of Sustainable Development Research 2020 4 no. 1 (2020): em0110. https://doi.org/10.29333/ejosdr/6343
Harvard
Megersa, S., and Feleke, S. (2020). Enzymatic Hydrolysis of Two-way Pretreated Sawdust of Eucalyptus globulus and Cupressus lusitanica. European Journal of Sustainable Development Research, 4(1), em0110. https://doi.org/10.29333/ejosdr/6343
MLA
Megersa, Shasho et al. "Enzymatic Hydrolysis of Two-way Pretreated Sawdust of Eucalyptus globulus and Cupressus lusitanica". European Journal of Sustainable Development Research, vol. 4, no. 1, 2020, em0110. https://doi.org/10.29333/ejosdr/6343